Services on Demand
Journal
Article
Share
Ciência & Educação
Print version ISSN 1516-7313On-line version ISSN 1980-850X
Ciência educ. vol.29 Bauru 2023 Epub Nov 06, 2023
https://doi.org/10.1590/1516-731320230048
ARTIGO ORIGINAL
The use of a meiosis concept inventory to identify alternative conceptions among Biological Science first-year students and prospective teachers
1Universidade Federal de Minas Gerais (UFMG), Instituto de Ciências Biológicas, Genética, Ecologia e Evolução, Belo Horizonte, MG, Brasil. Autora correpondente:
2Universidade Federal de Minas Gerais (UFMG), Faculdade de Educação, Belo Horizonte, MG, Brasil
Meiosis is regarded as a consistently challenging topic for students across various educational levels, constituting a domain within the field of genetics that has been extensively documented to elicit misunderstandings and alternative conceptions. The present study deals with elementary genetic concepts associated with meiosis among undergraduates in their first year (freshmen) and in their senior year (pre-service teachers). Overall performance on the inventory was similar for both groups, ranging from low to medium. The research revealed that students present alternative conceptions, particularly pertaining to the organization of gene alleles on chromosomes, the structure of chromosomes, cellular ploidy, DNA replication, and the products of meiosis. By conducting cognitive interviews with a chosen cohort of students, it was possible to analyze the way they formulated their responses and explanations. The results point to the need to emphasize the concepts discussed in this work in the teaching of genetics and cytology while concurrently devising novel instructional methodologies.
Keywords Ensino de genética; Ensino de biologia; Ciclo celular; Formação inicial do professor
A meiose é considerada um tópico persistentemente difícil para estudantes de diferentes níveis de ensino, sendo objeto de muitos relatos de entendimentos incorretos e concepções alternativas. Este é o primeiro trabalho a utilizar um Inventário de Conceitos de Meiose com estudantes de Biologia brasileiros para analisar a compreensão de conceitos genéticos elementares associados à meiose entre calouros e licenciandos veteranos. O desempenho geral no inventário foi semelhante para ambos os grupos, variando de baixo a médio. Observou-se que os estudantes compartilham concepções alternativas, especialmente sobre a disposição dos alelos gênicos nos cromossomos, estrutura dos cromossomos, ploidia celular, replicação do DNA e produtos da meiose. Entrevistas cognitivas com estudantes selecionados possibilitaram analisar como estes construíram suas respostas e explicações. Os resultados apontam para a necessidade de se enfatizar os conceitos discutidos neste trabalho no ensino de genética e citologia, bem como desenvolver novas estratégias de ensino.
Palavras-chave Ensino de genética; Ensino de biologia; Ciclo celular; Formação inicial do professor
Introduction
Despite the importance of Genetics and the role it plays in solving problems in the economic, environmental, social, and health sectors (CEZAR; GOMES; NIEDERAUER, 2020), there are numerous reports in the literature of misunderstandings of key concepts in this field, such as meiosis, which has been recognized as difficult for students to grasp for over 30 years (BROWN, 1990; KINDFIELD, 1994). Even to this day, it remains difficult for students to make associative links between meiosis and other topics of genetics, such as Mendelian inheritance (CARVALHO; SANTIAGO, 2020), whereas they continue to share alternative conceptions (AC) about fundamental concepts in the field of biology, from compulsory schooling to college, even after formal instruction (DIKMENLI, 2010; GUERRA; TAVARES; VILAS-BOAS, 2022; INFANTE-MALACHIAS et al., 2010; RODRÍGUEZ GIL; FRADKIN; CASTAÑEDA-SORTIBRÁN, 2019).
Since the 1970s, research in the field of Science Teaching has shown, with regard to the same specific content, that AC (understood here as prior understandings held by students that contradict scientific knowledge) are well-established and resistant to instruction, despite the fact that the students surveyed represent a wide range of cultural, personal, and educational backgrounds (DRIVER, 1989).
The study of AC has critically contributed to the advancement of the constructivist theory, as it recognizes that the acquisition of new knowledge stems from the modification and strenghtening of existing knowledge, with the learner assuming a central role in the knowledge-building process (MORTIMER, 1996). In this sense, AC should not be viewed solely as obstacles to be overcome or avoided, because, when triggered and subsequently activated, they can function as valuable learning resources to guide the teaching process and in metacognitive processes (LARKIN, 2012).
Conceptual inventories (CI) serve as valuable assessment tools for uncovering AC, while also providing guidance for instructional strategies and decision-making about program and curricula. The CI are well-established tools in the field, developed through a rigorous and comprehensive process involving experts and a large number of students (ADAMS; WIEMAN, 2011). For proof-of-concept purposes, these inventories typically bear resemblance to conventional multiple-choice assessments, with the inclusion of distractors, or 'incorrect' options, that are formulated based on research findings highlighting commonly held AC among students. The distractors serve to assess student's level of conceptual understanding and identify the point in which their understanding may have diverged or remained stagnant (GARVIN-DOXAS; KLYMKOWSKY; ELROD, 2007).
This research employed a modified version of the Meiosis Conceptual Inventory (MCI), which was created at the University of British Columbia in Canada (KALAS et al., 2013). In Brazil, there is currently a lack of published research utilizing the CI methodology in the field of Biology. Furthermore, international studies that made use of the MCI aimed to compare the effectiveness of different teaching approaches to the topic of meiosis in the classroom (NEWMAN; WRIGHT, 2017), whereas the purpose of this study was to investigate the understanding of concepts associated with the process of meiosis among Biological Sciences undergraduates at different points in the course and to identify and analyze possible AC shared by both groups of students: first-year and prospective teachers in their senior year of college.
While AC can be valuable teaching tools in specific situations, it is worth considering the continued reliance on them by aspiring educators, even after being exposed to the topic many times since early years of schooling and college education. This calls for thoughtful contemplation. In this situation, it is crucial to identify and understand these conceptions so that we can develop effective educational strategies to address and prevent any potential challenges that may arise for the teaching profession in the future. Likewise, conducting research with freshmen allows for the identification of the specific learning needs related to meiosis as they transition into higher education. By informing basic education instructors and university professors of these needs, it becomes feasible to consider teaching methods that promote the development of scientific understanding in the subject.
Methodological route
This study is quantitative and qualitative in nature. We gathered quantitative data by administering a questionnaire to 70 students enrolled in the Biological Sciences program at a federal university in Brazil. Additionally, we conducted qualitative data collection through interviews with a subset of 12 students. The study was presented to the Research Ethics Committee at the Federal University of Minas Gerais (CAAE: 88856618.6.0000.5149).
Students were divided into two groups: (i) Freshmen who had not previously taken the Genetics course, in which the topic of meiosis is covered; (ii) Seniors who had chosen to pursue teacher certification and who had already completed Genetics. The survey used in this study was created using the Meiosis Conceptual Inventory (MCI) (KALAS et al., 2013), which is made up of 17 questions particularly intended to assess undergraduate students' grasp of key concepts relevant to meiosis. These domains include ploidy, the relationship between DNA amount, chromosome number, and ploidy, the sequence of important events in meiosis, and chromosomal visualization. The MCI items are organized based on Bloom's Taxonomy of Cognitive Domains, a framework for categorizing learning goals. This framework arranges objectives in a hierarchical manner, with each level building upon the previous one. It starts with basic mental skills such as knowledge and understanding, and progresses towards more advanced skills such as applying and analyzing knowledge (BLOOM et al., 1956; FERRAZ; BELHOT, 2010).
To create the data collection instrument, twelve elements from the modified MCI were selected (SOUSA, 2020). The links to access the Informed Consent Form (ICM) and an explanatory video regarding the research were e-mailed to students enrolled in the Biological Sciences course. A total of 70 students voluntarily agreed to participate in the study, by sigining the Informed consent form. Administration of the form was online because of the COVID-19 pandemic in 2020. Microsoft Excel was used to analyze the data and determine the percentage of correct responses, incorrect answer patterns, and items where the distribution of chosen responses most closely matched the scientific consensus.
Following a check for data normality, the Mann-Whitney non-parametric test was used to assess the overall performance of the two independent samples of student participants on the revised MCI. Following a check for data normality, the Mann-Whitney non-parametric test was used to assess the overall performance of the two independent samples of student participants on the revised MCI. Fisher's exact test was used to compare the percentage of accurate answers by seniors and first-year students for each question. Version 8 of GraphPad Prism was used to create the statistical analyses and graphs.
From the 70 responders in the quantitative phase, twelve individuals qualified for interviews, which were conducted by video conference. The ICM was then used to pick seven items as the foundation for the interview script, from which alternative conceptions were found among the students, whose performance in the first stage of the survey was below 70%.
The cognitive interviewing technique was employed, wherein the survey items were administered to elicit supplementary verbal information from the test respondents. The verbal material obtained during the interviews encompassed the interviewees' explanations on the construction of their answers and their interpretations of the items. To gather this information, a probing methodology was employed. This method of inquiry involved using a combination of explicit questions, predetermined in a script, and an interviewer who took a proactive approach by directly asking the interviewees to elaborate on their answers (MEDEIROS et al., 2017).
Results and discussion
The analysis of the answers obtained with the adapted MCI from the 70 students participating in the study revealed that both groups, consisting of freshmen (n=25) and seniors (n=45), exhibited an overall average to low performance on the MCI (figure 1). Through the computation of average scores within each group, it was observed that first-years achieved an accuracy rate of 31.6%, while seniors attained 35.6%. Notably, according to the current curriculum structure consulted for courses such as Genetics I, Cell Biology, Embryology, and Genetics II, the seniors had already studied meiosis in college in greater depth than in high school. However, the analysis of each item revealed that there was no statistically significant difference (P>0.05) between the proportion of first-years and seniors who correctly answered each item. Interestingly, the similarity of the results found suggests that students in their first and last years of higher education have similar levels of understanding of the concepts covered.
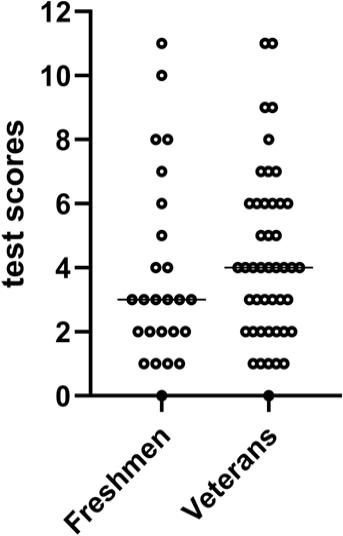
Note: Each circle symbolizes a different person, while the dash denotes the median. The general performance of the two independent samples of students was found to be similar (p=0.28) using the Mann-Whitney non-parametric test at a significance level of 5%.
Source: prepared by the authors.
Figure 1 Distribution of first-year students' (n=25) and seniors' (n=45) individual scores on the adapted MCI
Distribution of responses by item and categorization
The 12 items of the MCI were categorized utilizing a modified classification system as proposed by Smith e Knight (2012). The classification was performed by analyzing the frequency at which each alternative was chosen in each item, as well as the combinations of alternatives selected in multi-response format items. The objective was to ascertain the preference for specific distractors in comparison to the scientifically accepted alternative(s). (1) No apparent challenge: when a minimum of 70% of students provide accurate responses to a specific item; (2) Lack of a specific erroneous notion: when less than 70% of students exhibit a correct response to a given item, yet no particular incorrect alternative or specific combination of distractors was preferred over the other potential alternatives or combinations; (3) Common misconception: less than 70% of the students provide a correct response to a given item, while 30% show a preference for a specific distractor or a particular combination of distractors. The term Common Alternative Conception (CAC) was used to describe an incorrect answer chosen by at least 30% of the pupils.
Analysis of Common Alternative Conceptions (CAC)
Figure 2 displays the performance of the two sample groups, namely first-year students and seniors, on each of the 12 items in the adapted MCI. The observed slight superiority in the performance of newcomers compared to more experienced students, specifically in items 1, 2, 3, 4, and 9 (which pertain to ploidy and number of chromosomes), did not yield statistically significant results (P>0.05).
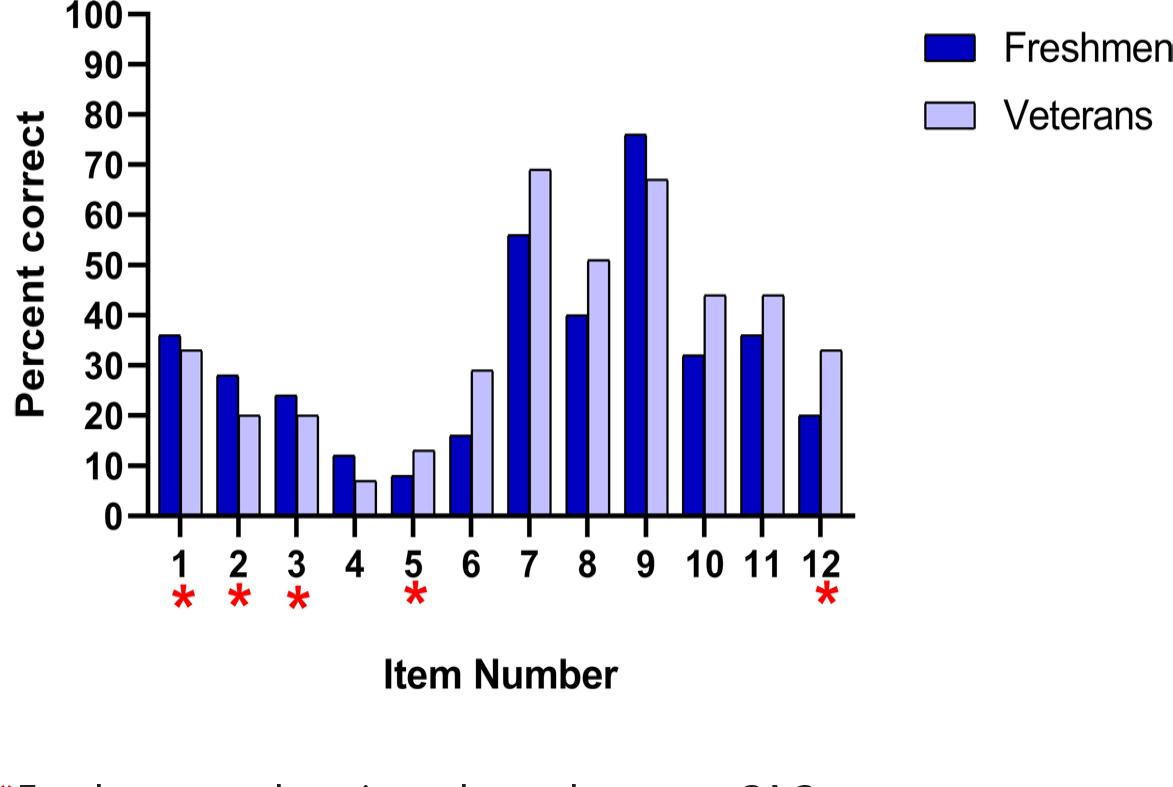
*Freshmen and seniors share the same CAC.
Source: prepared by the authors.
Figure 2 Performance of first-years and seniors on the 12 selected items of the MCI (n=70 students)
The items that have been indicated with an asterisk are the ones where students in both groups displayed a preference for certain distractors. These distractors represent the Common Conceptual Schema (CCS), which are further elaborated in table 1.
Table 1 Frequency of choice of alternative conceptions identified in category 3 items
Item | Common Alternative Conceptions (CAC) | Frequency of CAC selection | |||
---|---|---|---|---|---|
RS | MR | Freshmen | Seniors | ||
1 | x | The absolute number of chromosomes determines cell ploidy. | 40% | 60% | |
2 | x | (i) Haploid cells cannot have chromosomes composed of sister chromatids; (ii) Confusion between the terms sister chromatids and homologous chromosomes. | 60% | 71% | |
3 | x | A cell is considered diploid if its chromosomes are made up of sister chromatids. | 56% | 71% | |
4 | x | Two sister chromatids are two chromosomes. | - | 31% | |
5 | x | Understands the relationship between chromosomes and sister chromatids, but does not relate it with DNA replication. | 48% | 47% | |
7 | x | DNA is single stranded before replication; the diagram is drawn after replication. If there are two complementary strands, the DNA becomes double-stranded. | 40% | - | |
11 | x | Homologous chromosomes appear paired in both mitosis and meiosis metaphase. | 40% | - | |
12 | x | Confusion between meiosis and mitosis: the idea that, genetically, a gamete looks like a post-mitotic cell. | 52% | 53% |
Note: 30% or more freshmen (n=25) and seniors (n=45) preferentially selected a specific incorrect answer, called CAC.
RS: Single-answer multiple-choice items (only one of four answer options may be chosen).
MR: multiple-response multiple-choice items (any number can be chosen from four answer options).
Source: prepared by the authors.
Despite being at different stages of their undergraduate studies, first-year and senior-year students shared five CAC (indicated by an asterisk in figure 2 and elaborated in table 1). The alternative conceptions identified among first-year students may have originated in their prior education and may or may not undergo modifications to align with scientific conceptions. However, the seniors, students who possess a theoretically more profound understanding of biological topics, maintain comparable levels of academic competence, despite their repeated encounters with the subject in formal education and even after receiving instruction at the higher education level from different instructors utilizing various teaching methodologies.
The graphs depicted in figure 3 illustrate the instances where freshmen and seniors exhibited a shared inclination towards the same distractors. Each graph, corresponding to a specific item, displays the correct option denoted by an asterisk and the preferred distractors. In item 1, for instance, the accurate choice is letter B*, while the distractor commonly indicated by both samples was letter A. The items encompass fundamental concepts pertaining to meiosis, including ploidy, chromosome structure, and visual identification of cells following meiosis.
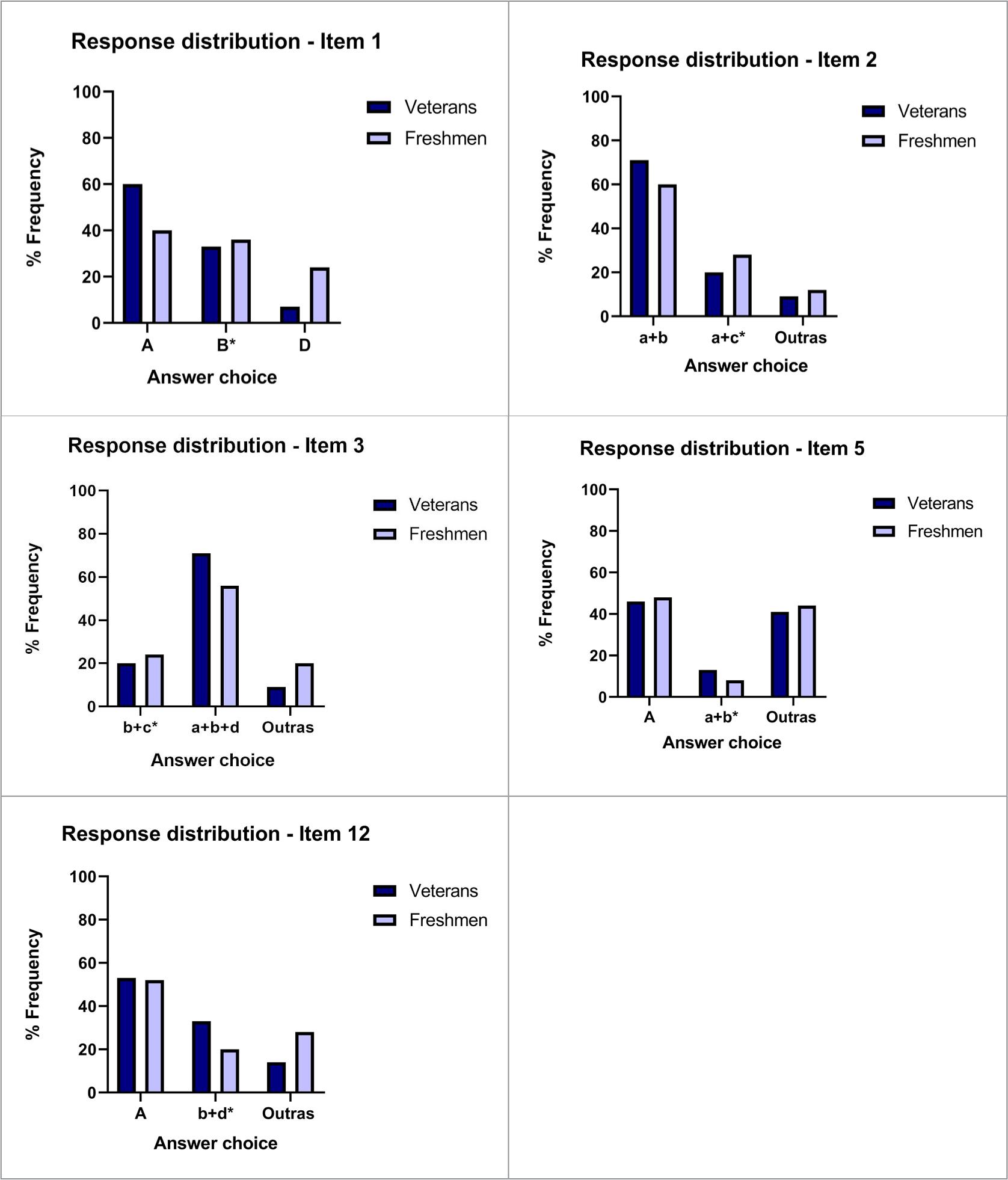
Note: The right response is indicated by an asterisk (*). The following topics are covered in each item: ploidy (items 1, 2, and 3), chromosome structure (item 5), and pictorial identification of meiotic products (item 12). Source: prepared by the authors.
Figure 3 Response choice to items 1, 2, 3, 5, and 12 were distributed differently among freshmen (purple) and seniors (blue)
The primary AC identified with the concept of ploidy is the notion that cell ploidy can vary based on the structure of chromosomes. Kindfield (1994), in a study conducted at the University of California, and Guerra, Tavares, and Vilas-Boas (2022), in a study conducted at the same institution that is the focus of this research, discovered that a similar conception is shared by students. In this line of reasoning, non-replicated chromosomes are derived from haploid cells, while replicated chromosomes originate from diploid cells. Additionally, it is believed that the total count of chromosomes is indicative of a cell's ploidy. Consequently, diploid cells consistently possess a greater number of chromosomes compared to haploid cells, irrespective of the specific species under consideration.
Identification of AC and their inclusion in the Ploidia conceptual category were associated with a decline in students’ performance as more advanced cognitive skills were demanded in items 1, 2, and 3 (figure 2). The student’s performance on item 1, which falls into the comprehension category according to Bloom's taxonomy, was superior, despite not reaching the satisfactory threshold of 70%. This outcome could be explained by the fact that the item only asks the student to comprehend and explain the concept of cell ploidy. On the other hand, the students performed less well on items 2 and 3 from the Application category (level III in Bloom’s taxonomy), which asks them to apply their understanding of ploidy to novel circumstances - in this case, diagrammatic representations. Although item 4 falls within the same conceptual category as items 1, 2, and 3, its ploidy aspect posed a significant challenge for students in comparison to the other items. Additionally, multiple alternative concepts were identified for item 4, as depicted in figure 2. This phenomenon can be elucidated by the requirement of a higher cognitive skill, specifically analysis, as outlined in Bloom’s Taxonomy. The understanding of ploidy is necessary for comprehending the item, as it entails the capacity to dissect the content into discrete components and discern their interconnectedness. Thus, the student should comprehend how the concept of ploidy is linked to other concepts, including the count of chromosomes, the structure of chromosomes (whether sister chromatids are present or absent), and the organization of gene alleles. In addition, analytical skills necessitate familiarity with the ultimate structure of the subject under study, such as the accurate portrayal of a diploid and haploid cell (BLOOM et al., 1956; FERRAZ; BELHOT, 2010).
Cell ploidy was unquestionably a difficult concept for both novice and advanced students. The undergraduates achieved varying levels of cognitive proficiency about this concept. While many possess a theoretical understanding of the term, they struggle with its practical application in novel contexts or its integration with other concepts in the field of Genetics. In Bloom's Taxonomy of Cognitive Objectives, for a student to advance to a new category in the hierarchy of cognitive skills, they must have performed adequately in the previous category, as new skills rely on previously acquired capacities (BLOOM et al., 1956; FERRAZ; BELHOT, 2010).
The item that exhibited the second lowest performance among the students was item 5, which deals with chromosome structure. Notably, both first-year students and seniors demonstrated a percentage of correct answers below 15% for this item, as illustrated in figure 2. Some students found it difficult to distinguish the concepts chromosomes and chromatids; another portion showed that they understood the relationship between chromosomes and chromatids, but did not establish a relationship between the origin of chromatids, knowledge at the molecular level (DNA replication), and chromosome structure or were unaware of this phenomenon of interphase (47% of seniors and 48% of freshmen). These results align with the findings in the existing literature, spanning from earlier to more recent studies conducted with students of diverse backgrounds. In Brown's (1990) study, a total of 614 meiosis representations created by British students were analyzed. It was observed that approximately 50% of the participants in the survey displayed incorrect depictions of chromosomes following DNA replication. These inaccuracies included the portrayal of chromosomes with sister chromatids of varying colors, non-duplicated chromosomes, or chromosomes with four chromatids each. In the study conducted by Guerra, Tavares, and Vilas-Boas (2020), it was observed that meiosis diagrams created by Brazilian teachers in training exhibited a common error. Specifically, the most prevalent mistake was the omission of duplicated chromosomes during interphase, rather than after this phase. Additionally, these diagrams often depicted sister chromatids with heterozygous alleles during interphase, disregarding the fact that sister chromatids are identical at this stage. It is apparent that a notable portion of students lack comprehension regarding DNA replication and its connection to meiosis, even though this biological process is imperative for cellular division to occur.
In relation to the outcome of the meiotic process, roughly half of first-year or senior students exhibit confusion between the products of mitosis and meiosis, as indicated in item 12. It is commonly assumed by students that gametes bear resemblance to postmitotic cells. This perception may arise from the commonly circulated discourse that meiosis II bears resemblance to mitosis, which can cause confusion regarding the progeny cells produced by both processes.
It is widely acknowledged that the conceptions that students possess prior to entering the classroom regarding scientific phenomena play a crucial role in the process of learning. These pre-existing notions allow students to articulate and compare their previous ideas with the scientific concepts being taught. In this context, it is important to refrain from reducing AC to misconceptions that need to be eliminated or replaced. Instead, one can explore and utilize them as valuable teaching resources in the advancement of scientific conceptions (LARKIN, 2012). However, the preference for the same alternative conceptions (AC) among novice and veteran undergraduate students indicates that certain conceptual frameworks have become deeply ingrained and may exhibit significant resistance to instructional interventions. AC, initially serving as valuable tools in educational settings, can subsequently compete with scientifically accepted concepts. Their persistance within the cognitive framework of aspiring educators has the potential to perpetuate a cycle of disseminating these misconceptions in the classroom, hindering the progress of professional development and instructional practice.
Cognitive interviewing
The study involved conducting interviews with 12 students, providing an opportunity to examine the cognitive processes that influenced their decision-making in the MCI. The participants were provided with the items and subsequently instructed to restate their responses while providing a rationale for their selections to the interviewer. Upon conducting an analysis of the transcribed statements, it was observed that four distinct categories emerged. (1) The organization of genetic alleles. (2) The structure of chromosomes. (3) The process of DNA replication. (4) The products of meiosis. To improve the clarity of the responses, a presentation of the constituent elements that make up each category is provided in Figures 4 to 6. The verbal expressions of the students are emphasized with italics, with each one being denoted by the letter E followed by a number.
Category 1: The organization of genetic alleles
In item 4, the AC identified in the quantitative stage was that sister chromatids are considered as separate chromosomes. According to the students’ understanding, a duplicated chromosome refers to the presence of two chromosomes. During the interview stage, new alternative conceptions were identified, including the notion that Aa genotypes indicate the presence of the A allele on one sister chromatid and the a allele on the other sister chromatid, without requiring crossingover. In this scenario, when students observed a duplicated chromosome with an A allele on both sister chromatids and its corresponding counterpart with a a allele on both chromatids, as depicted in the diagrams of letters B and D (figure 4), some students mistakenly believed that the genotype was duplicated. They described the genotype as AAaa for this gene locus instead of Aa:
E4 (Item 4, figure 4): I believe it's the letter A [...]. In [letter] B and [letter] D they put the AA alleles on the same chromosome, as if the information was duplicated like that [...] the chromosomes with the two strands and on those two strands the alleles are the same, AA, and there's another strand with aa, that doesn't make much sense to me.
Another AC found in this category was that Aa genotypes indicate that dominant and recessive alleles cannot coexist on the same chromosome. Because of this, dominant alleles A, B, and D should be on the same chromosome and recessive alleles a, b, and d on the other, as shown in the diagram - letter B (figure 4):
E8 (Item 4, figure 4): [...] I believe that one of the chromosomes contains these dominant alleles while the other would contain these recessive alleles.
Elementary genetic concepts are commonly introduced in classes about Mendel’s Laws, where the concept of genetic alleles is typically discussed. Nonetheless, teachers do not always teach this term in greater detail beyond conceptualization, such as the proper arrangement of alleles on chromosomes (GUERRA; TAVARES; VILAS-BOAS, 2022). One example is the utilization of Punnett's square, in which students arrange the alleles on a chart to display the genotype of the gametes of the parents and offspring. However, the precise positioning of these alleles within the homologous chromosomes, as well as the intricate mechanisms involved in the process of meiosis, are occasionally overlooked. Therefore, students may develop a tendency towards mechanistic thinking, lacking the ability to establish connections among pertinent concepts such as genotype, gene locus, sister chromatids, homologous chromosomes, DNA replication, and alleles.
In an analysis of diagrams of meiosis completed by undergraduates in Biological Sciences, Guerra, Tavares, and Vilas-Boas (2020) identified AC with respect to heterozygosity and alleles. The students depicted cells containing dominant and recessive alleles that were arranged on a single chromosome, without any instances of permutation. The reasoning in this study is akin to that of the students because, in both instances, the researchers failed to consider the fundamental concept that DNA replication results in the formation of identical sister chromatids. Additionally, it is important to note that the allelic forms are situated at the same gene locus in the homologous chromosomes, rather than on the sister chromatids.
In addition to a lack of knowledge of gene alleles between homologues, students may experience conceptual difficulties due to the fact that the language of genetics is characterized by extensive terminology and brings together abstract concepts that are sometimes used interchangeably, such as genes, alleles, and loci. One example is the common usage of the term gene to refer to a specific genetic allele, such as the gene associated with Duchenne muscular dystrophy. Furthermore, if the student is familiar with the concept of allele, they may not have fully grasped the representation of genetics at the molecular and microscopic levels through diagrams and symbols.
Category 2: The structure of chromosomes
Regarding the relationship between chromosomes and sister chromatids, some interviewees believe that ‘true’ chromosomes consist of two chromatids, whereas unreplicated chromosomes are merely chromatids, and thus should not be considered individual chromosomes:
E9 (Item 8, figure 5): It would only be [letter] C, right?
Entrevistadora: Do you think there are how many chromosomes here [in letter B]? E9: There won't be any, there will be chromatids.
This idea that chromosomes will always be made up of sister chromatids during cell division was discovered to be shared by 18% of seniors in the quantitative stage and is consistent with the study by Dikmenli (2010), which was carried out with 124 Turkish undergraduates. According to the author, this concept had not been previously reported in the literature. Afterwards, the same conception was found among 25% of a sample of 193 Canadian university students (KALAS et al., 2013).
Guerra, Tavares, and Vilas-Boas (2022) note that it is commonly assumed in biology teaching that chromosomes must always be composed of sister chromatids. This occurs because chromosomes are typically defined in terms of highly compressed structures, specifically the duplicated metaphase chromosomes that can be seen using an optical microscope. It is thus important to emphasize to students that both duplicated and nonduplicated forms of chromosome representation are equally valid. However, the correct representation depends on the specific moment in the cell cycle when the cell is located.
Additionally, some of the subjects of the interviews were unable to distinguish between the terms chromosomes and sister chromatids. Approximately 30% of first-year students or seniors commonly believe that each chromatid in a pictorial representation is considered a distinct chromosome:
E3 (Item 8, figure 5): For this question, I’d choose both letter A and letter B, as, in letter A, for example, there are eight chromosomes, even though they’re homologous, four of them are duplicates. In option B, the chromosomes are not duplicated. It is a haploid cell, but there are still eight chromosomes. In letters C and D, they got a total of 16 chromosomes.
E4 (Item 8, figure 5): I’d go with marking [letters] A and B, keeping in mind what is a chromosome. A chromosome is basically the ribbon, a ribbon-like thing. So, like, if there are eight chromosomes, you just gotta check out which [cells] have eight ribbons, and these are in [letters] A and B.
In the findings of Kalas et al. (2013), the developers of the ICM, the distinction between the two concepts was identified as one of the main difficulties encountered by the respondents. According to a study conducted by Infante-Malachias et al. in 2010, freshmen and senior students enrolled in the biology course at the University of São Paulo (USP) showed difficulty distinguishing between homologous chromosomes and sister chromatids when asked to draw or identify them. Many university students identified the separate chromatids by dividing the short and long chromosome arms. This approach resulted in a break within each chromatid and the formation of V-shaped figures. Moreover, Dikmenli (2010) discovered through drawings and interviews that around 30% of undergraduate biology students believed that homologous chromosomes and sister chromatids had identical meanings.
There is a need to emphasize the difference between the two concepts in classes on the subject because, although they are still terms dealt with in basic education, alternative conceptions persist in higher education. Differentiating between the two concepts may be challenging due to the varying visual representations of chromosomes that students encounter when studying Genetics and Cytology. These representations can include the shape of a ‘X’ or other forms, as shown in item 5 (figure 5). Because of the similarity in spelling and phonetics, which adds up to the lexical density of genetics, it is also possible to understand why these concepts are confusing.
A common source of confusion among students pertains to the notion that the composition of chromosomes shows variability depending on the ploidy level of the cell. This alternative conception aligns with the results obtained from the quantitative phase of this investigation, which were supported by 71% of seniors and 60% of freshmen:
E3 (Item 4, figure 4): So, at this one, my first move would be to rule out alternative C, because I reckon that’s a haploid cell, and it's claiming that the plant is diploid.
Interviewer: Why do you reckon it's haploid?
E3: Considering that just one little stick is there, I assume it's just one chromosome being depicted, you know? Because it's not duplicated. [...] I reckon that [letters] B and D represent the diploid chromosomes, from a diploid cell.
Likewise, Guerra, Tavares, and Vilas-Boas (2022) found that undergraduates understand that n refers to cells with single chromosomes and 2n to those with duplicated chromosomes. With the same reasoning, Finnish university students associate the state of the chromosomes - replicated or not - with the concepts of diploidy and haploidy, respectively, even after lectures on the subject (MURTONEN; NOKKALA; SÖDERVIK, 2018). Similar AC have been documented in older literature (KINDFIELD, 1994), indicating that these conceptions persist among students from various cultural backgrounds and educational levels.
In this study, three potential factors were examined that could potentially influence the development and longevity of alternative conceptions regarding ploidy and chromosome structure. One idea held by some students is that DNA replication increases the cell's ploidy, as stated by student E4:
E4 (Item 6): It's because if I'm thinking about replication, which is going to give rise to two daughter cells, a cell that is 2n would have to be, temporarily, 4n, the mother cell, so that the two daughters, each, are 2n.
Therefore, prior to DNA replication, the student identifies a haploid cell with a single chromosome. After replication, the student notices a diploid cell with duplicated chromosomes. The second reason for confusion lies in the distinction between chromatids and homologous chromosomes. In a diploid cell, the presence of sister chromatids, rather than homologous chromosomes, is what defines its characteristics. AC may have a connection to the origins of certain words. For instance, the term diploid is derived from the Greek word diplòos, which means double. Similarly, the term haploid is derived from the Greek word haplo, which means single. This association between the terms diploid and haploid and the concepts of double and single chromosomes, respectively, may be influenced by these etymological origins.
In relation to the categorization of chromosomes based on the location of the centromere, there exists a perspective among certain students positing that telocentric chromosomes are incomplete, implying the absence of certain segments:
E9 (Item 8, figure 5): [...] there [student points to letter D] they [the chromosomes] are incomplete, right? Some of them.
This conception indicates a limited understanding of the various types of chromosomes. The students may be primarily acquainted with the typical depiction of metacentric chromosomes. In a study conducted by Rodríguez Gil, Fradkin, and CastañedaSortibrán (2019), it was observed that students at a university in Mexico did not include telocentric and acrocentric chromosomes in their drawings, even though they were specifically instructed to do so. The explanation for this result is provided by the authors, who highlight that metacentric chromosomes are frequently observed and studied. These chromosomes are immobilized during the metaphase of mitosis and exhibit a classical ‘X’ shape. Additionally, the metacentric human chromosomes are the largest and most visible, which accounts for their widespread representation.
Finally, a dissociation was identified between the representation of duplicated chromosomes and the underlying molecular process, DNA replication:
E6 (Item 5, figure 5): The chromosome is not duplicated, it only has its two sister chromatids.
E9 (Item 5, figure 5): That one I think would be the letter A, two sister chromatids [...]. To me, it does not represent a replication process.
There is a disconnection between two interconnected fields of biology, namely Molecular Biology and Genetics. This result was also observed in the quantitative stage among approximately 50% of the students, providing confirmation for the initially formulated hypothesis. Guerra, Tavares, and Vilas-Boas (2022) have reported challenges in visualizing microscopic structures, such as chromosomes, and establishing their connection to the underlying molecular processes. Lewis, Leach, and Wood-Robinson (2000) highlight a significant challenge faced by students regarding the inherent connection between fundamental genetic structures, such as the physical relationship between genes, DNA, and chromosomes. This challenge is made worse by the unclear use of terminology when subjects are taught in separate parts and when the connection between ideas is not highlighted. According to Chu and Reid (2012), one obstacle to learning Genetics is the requirement to navigate various levels of biological organization, including molecular, microscopic, symbolic, and phenotypic levels. Reasoning about concepts and processes that occur at multiple levels simultaneously can potentially lead to mental overload for students.
Category 3: The process of DNA replication
Item 6: In a eukaryotic cell, DNA replication results in an increase in: (a) amount of DNA in that cell. (b) number of chromosomes in that cell. (c) number of DNA molecules in that cell. (d) ploidy of that cell (e.g., from 2n to 4n). (KALAS et al., 2013).
The hypothesis formulated in the quantitative step, regarding the relationship between DNA replication and cell division, is that students do not remember or are unaware of the process by which sister chromatids are formed. In this qualitative step, a new alternative conception was identified, which suggests that DNA replication results in the formation of a new chromosome:
E2 (Item 6): [DNA replication] increases the number of molecules and the number of chromosomes. The number of DNA molecules, because they’re going to take one as a template to form the other, so, the number of DNA molecules will double. I think it’s going to do that with all the material, right? I think the number of chromosomes will double as well.
The alternative conception mentioned above may arise from the use of different mathematical terms that are used interchangeably in the field of Genetics. For instance, DNA replication is often referred to as chromosome duplication, which can cause confusion among students. This ambiguity in terminology may lead students to believe that DNA replication always leads to an increase in the number of chromosomes. Additionally, students may mistakenly think that DNA replication is a necessary process for the mother cell to accurately distribute its genetic material to the daughter cells. The conception mentioned was also discovered by Kalas et al. (2013) in a study involving 40% of the students who were involved in the development of the ICM at the University of British Columbia. It is noteworthy that some students may lack awareness of the phase that occurs before mitosis and meiosis, along with its characteristics, significance, and molecular and microscopic implications.
Category 4: The products of meiosis
In terms of meiotic products, some students contend that gametes are like mitotic cells in that they contain the same genetic material as the mother cell. This idea aligns with the data that was interpreted during the quantitative step among approximately 50% of all students:
E3 (Item 12, figure 6): I think I would only mark letter A in this one [...]. In letter B and letter D, I think we’re missing chromosomes. They’re representing some of the chromosomes of the mother cell, but since it said that it went through regular meiosis, I’m thinking that it might be lacking one chromosome or something. The letter A is one of the representations of the potential daughter cells.
The confusion between mitosis and meiosis was identified by Gil, Fradkin e CastañedaSortibrán (2018). These researchers observed that Mexican university students created similar diagrams of meiosis II and mitosis in their cell division illustrations. In a study conducted by Etobro and Banjoko (2017), a multiple-choice genetics exam was used to evaluate 120 Nigerian educators. The results showed that 84% of the participants correctly understood that meiosis in a mother cell leads to the formation of identical daughter cells. This conception can be attributed to the similarity between the two division processes and the idea, often disseminated during teaching and information sources, that mitosis is equivalent to meiosis II. This association has the potential to cause confusion among students regarding the outcomes of the two processes, potentially leading them to mistakenly believe that gametes resemble post-mitotic cells.
One potential approach to enhance comprehension of the meiosis process is to employ a variety of teaching strategies, moving away from relying solely on a single method, such as the traditional approach that emphasizes knowledge transmission and memorization. Implementing a plural methodological proposal is important in education, as students possess diverse cognitive, motivational, and emotional backgrounds. These differences may lead to individual preferences regarding teaching styles (LABURÚ; ARRUDA; NARDI, 2003).
There are several proposals in the scientific literature for additional ways of teaching meiosis. Two examples include the combine and recombine with dominoes approach (GUIMARÃES et al., 2008) and the Meiosis on paper method (VILAS-BOAS; TAVARES, 2022). These approaches utilize low-cost and easily prepared models to visually represent each stage of the meiotic process, including the representation of different alleles. These activities provide students with an opportunity to explore the connection between meiosis and Mendel’s Laws, sexual reproduction, genetic variability, and fundamental genetic concepts like genotype, homologous chromosomes, sister chromatids, and ploidy.
Final remarks
In Brazil, the use of inventories has received little attention in the field of biology. This study is a pioneering effort to utilize a meiosis concept inventory along with Brazilian students. The analyses indicate that the inventory was an effective tool for identifying shared conceptual understanding between first-year students and seniors in a Biological Sciences course. The MCI also supported cognitive interviews, which were found to be helpful in analyzing the understanding of meiosis among the undergraduates in the study. The MCI could potentially be applied in other studies with comparable objectives, such as those involving students from different academic disciplines or even experienced educators. It can also be utilized to evaluate the effectiveness of implementing new teaching methodologies in the subject and to provide guidance for curricular reforms.
The analysis of the MCI and cognitive interviews conducted in this study revealed that first-year students and trainee teachers share similar AC on elementary concepts associated with meiosis. These concepts include cell ploidy, chromosome structure and number, the arrangement of gene alleles, DNA replication, and the products of meiosis. Thus, it is important to consider alternative approaches to teaching and learning in well-established fields of Genetics, such as cell division and Mendelian genetics. This work suggests that teachers should prioritize the concepts discussed here, regardless of whether they have been taught in primary or higher education. The teaching plan should include a more comprehensive exploration of students' prior knowledge. This will enable students to better understand any existing conceptual frameworks they may have and utilize them as a foundation for acquiring new knowledge.
Our findings have the potential to stimulate discourse on these concepts among educators and learners in Brazil and worldwide, as well as to facilitate implementing pedagogical measures, such as novel educational approaches and instructional resources that effectively highlight the AC, as observed in this study and elsewhere.
Acknowledgements
The authors express their gratitude to the National Council for Scientific and Technological Development (CNPq) and the Coordination for the Improvement of Higher Education Personnel (CAPES) for providing financial support.
REFERENCES
ADAMS, W. K.; WIEMAN, C. E. Development and validation of instruments to measure learning of expert-like thinking. International Journal of Science Education, Abingdon, UK, v. 33, n. 9, p. 12891312, 2011. Doi: https://doi.org/10.1080/09500693.2010.512369. [ Links ]
BLOOM, B. S.; ENGELHART, M. D.; FURST, E. J.; HILL, W. H.; KRATHWOHL, D. R. Taxonomy of educational objectives. New York: David Mckay, 1956. [ Links ]
BROWN, C. R. Some misconceptions in meiosis shown by students responding to an advanced level practical examination question in biology. Journal of Biological Education, Philadelphia, v. 24, n. 3, p. 182-186, 1990. Doi: https://doi.org/10.1080/00219266.1990.9655138. [ Links ]
CARVALHO, H. F.; SANTIAGO, S. A. A fragilidade do ensino da meiose. Ciência & Educação, Bauru, v. 26, p. 1-15, 2020. Doi: https://doi.org/10.1590/1516-731320200025. [ Links ]
CEZAR, K. G.; GOMES, L. I.; NIEDERAUER, C. A. P. Análise e organização da informação nas instituições: definição de temas prioritários em biotecnologia e genética. Revista Ibero-Americana de Ciência da Informação, Brasília, v. 13, n. 1, p. 167-183, 2020. Doi: https://doi.org/10.26512/rici.v13.n1.2020.24753. [ Links ]
CHU, Y. C.; REID, N. Genetics at school level: addressing the difficulties. Research in Science and Technological Education, Abingdon, UK, v. 30, n. 3, p. 285-309, 2012. Doi: https://doi.org/10.1080/02635143.2012.732059. [ Links ]
DIKMENLI, M. Misconceptions of cell division held by student teachers in biology: a drawing analysis. Scientific Research and Essays, Nigeria, v. 5, n. 2, p. 235-247, 2010. Disponível em: https://tinyurl.com/mrx96547. Acesso em: 10 ago. 2022. [ Links ]
DRIVER, R. Students’ conceptions and the learning of science. International Journal of Science Education, Abingdon, UK, v. 11, n. 5, p. 481-490, 1989. Doi: https://doi.org/10.1080/0950069890110501. [ Links ]
ETOBRO, A. B; BANJOKO, S.O. Misconceptions of genetics concepts among pre-service teachers. Global Journal of Educational Research, Calabar, Nigeria, v. 16, n. 2, p. 121-128, 2017. Doi: https://doi.org/10.4314/gjedr.v16i2.6. [ Links ]
FERRAZ, A. P. C. M.; BELHOT, Renato V. B. Taxonomia de Bloom: revisão teórica e apresentação das adequações do instrumento para definição de objetivos instrucionais. Gestão & Produção, São Carlos, v. 17, n. 2, p. 421-431, 2010. Doi: https://doi.org/10.1590/S0104-530X2010000200015. [ Links ]
GARVIN-DOXAS, K.; KLYMKOWSKY, M; ELROD, S. Building, using, and maximizing the impact of concept inventories in the biological sciences: report on a national science foundationsponsored conference on the construction of concept inventories in the biological sciences. CBE Life Sciences Education, Bethesda, US, v. 6, p. 277-282, 2007. Doi: https://doi.org/10.1187/cbe.07-05-0031. [ Links ]
GUERRA, L. F.; RIBEIRO, R. C.; TAVARES, M. L.; VILAS-BOAS, A. Desenvolvimento de um método de análise de diagramas sobre meiose que auxilia avaliar erros de compreensão sobre a divisão celular. Experiências em Ensino de Ciências, Campo Grande, v. 15, n. 3, p. 108-123, 2020. Disponível em: https://fisica.ufmt.br/eenciojs/index.php/eenci/article/view/771. Acesso em: 15 ago. 2022. [ Links ]
GUERRA, L. F.; TAVARES, M. L.; VILAS-BOAS, A. Persistência de concepções alternativas sobre meiose no ensino de genética entre licenciandos de ciências biológicas. Dynamis, Blumenau, v. 28, n. 1, p. 107-126, 2022. Doi: https://doi.org/10.7867/1982-4866.2022v28n1p107-126. [ Links ]
GUIMARÃES, M. N. K.; OLIVEIRA, S. F.; AKIMOTO, A.; HIRAGI, C.; BARBOSA, L. S.; ROCHA, D. M. S.; CORREIA, A. Combinar e recombinar com os dominós. Genética na Escola, São Paulo, v. 3, n. 2, p. 1-7, 2008. Doi: https://doi.org/10.55838/1980-3540.ge.2013.164. [ Links ]
INFANTE-MALACHIAS, M. E.; PADILHA, I. Q. M.; WELLER, M.; SANTOS, S. Comprehension of basic genetic concepts by Brazilian undergraduate students. Revista Electrónica de Enseñanza de las Ciencias, Vigo, v. 9, n. 3, p. 657-668, 2010. Disponível em: http://reec.uvigo.es/volumenes/volumen9/ART9_Vol9_N3.pdf. Acesso em: 17 ago. 2022. [ Links ]
KALAS, P.; O’NEILL, A.; POLLOCK, C.; BIROL, G. Development of a meiosis concept inventory. CBE Life Sciences Education, Bethesda, US, v. 12, n. 4, p. 655-664, 2013. Doi: https://doi.org/10.1187/cbe.12-10-0174. [ Links ]
KINDFIELD, A. C. H. Understanding a basic biological process: Expert and novice models of meiosis. Science Education, Hoboken, US, v. 78, n. 3, p. 255-283, 1994. Doi: https://doi.org/10.1002/sce.3730780308. [ Links ]
LABURÚ, C. E.; ARRUDA, S. M.; NARDI, R. Pluralismo metodológico no ensino de ciências. Ciência & Educação, Bauru, v. 9, n. 2, p. 247-260, 2003. Doi: https://doi.org/10.1590/S1516-73132003000200007. [ Links ]
LARKIN, D. Misconceptions about “misconceptions”: preservice secondary science teachers’ views on the value and role of student ideas. Science Education, Hoboken, US, v. 96, n. 5, p. 927-959, 2012. Doi: https://doi.org/10.1002/sce.21022. [ Links ]
LEWIS, J.; LEACH, J.; WOOD-ROBINSON, C. Chromosomes: the missing link - young people’s understanding of mitosis, meiosis, and fertilisation. Journal of Biological Education, Philadelphia, US, v. 34, n. 4, p. 189-199, 2000. Doi: https://doi.org/10.1080/00219266.2000.9655717. [ Links ]
MEDEIROS, L.; COSTA, D. O.; GUEDES, A. T.; JALOTO, A. Aprendendo com as respostas dos estudantes a itens dos testes das avaliações de larga escala: lições do sistema nacional de avaliação da educação básica. Revista Com Censo, Brasília, v. 4, n. 4, p. 14-24, 2017. [ Links ]
MORTIMER, E. F. Construtivismo, mudança conceitual e ensino de ciências: para onde vamos? Investigações em Ensino de Ciências, Porto Alegre, v. 1, n. 1, p. 20-39, 1996. Disponível em: http://www.if.ufrgs.br/public/ensino/N1/2artigo.htm. Acesso em: 18 ago. 2022. [ Links ]
URTONEN, M.; NOKKALA, C.; SÖDERVIK, I. Challenges in understanding meiosis: fostering metaconceptual awareness among university biology students. Journal of Biological Education, Philadelphia, US, v. 54, n. 1, p. 3-16, 2018. Doi: https://doi.org/10.1080/00219266.2018.1538016. [ Links ]
NEWMAN, D. L.; WRIGHT, L. K. Meiosis: a play in three acts, starring DNA sequence. CourseSource, US, v. 4, 2017. Doi: https://doi.org/10.24918/cs.2017.9. [ Links ]
RODRÍGUEZ GIL, S. G.; FRADKIN, M; CASTAÑEDA-SORTIBRÁN, A. N. Conceptions of meiosis: misunderstandings among university students and errors. Journal of Biological Education, Philadelphia, US, v. 53, n. 2, p. 1-14, 2019. Doi: https://doi.org/10.1080/00219266.2018.1469531. [ Links ]
SMITH, M. K.; KNIGHT, J. K. Using the genetics concept assessment to document persistent conceptual difficulties in undergraduate genetics courses. Genetics, Cary, v. 191, n. 1, p. 21-32, 2012. Doi: https://doi.org/10.1534/genetics.111.137810. [ Links ]
VILAS-BOAS, A.; TAVARES, M. L. O essencial da genética no ensino médio: guia de atividades. Belo Horizonte: Proex UFMG, 2022. Disponível em: http://www.icb.ufmg.br/neducom/ciencia-na-escola. Acesso em: 27 set. 2022. [ Links ]
Received: April 28, 2023; Accepted: July 14, 2023